Profiles in Diagnostics: A Hot Pursuit to Understand ICE
Kathreen Thome studies how particles travel through fusion plasmas using high frequency magnetic fluctuations known as ion cyclotron emission (ICE).
The tokamak approach to magnetic confinement fusion uses a toroidal solenoid to confine high temperature plasmas. With peak plasma temperatures above 100 million degrees Celsius, no physical measurements are possible beyond the very edge of the devices. The unique diagnostic challenges of tokamak experiments are being overcome by a talented group of early career researchers keen to develop fusion energy. Today’s profile follows the work of Kathreen Thome as she investigates the relationship between MHz magnetic fluctuations and the trajectories of energetic ion species in the DIII-D National Fusion Facility.
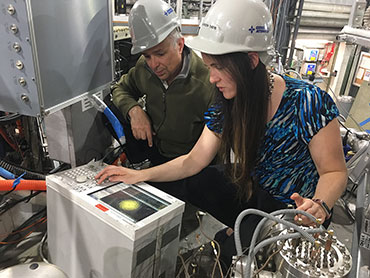
The ultimate goal of the tokamak design is to house a plasma that produces so much nuclear fusion through the reaction D + T = alpha + neutron that the excess energy both generates electricity and keeps the plasma hot enough to continue high fusion rates without additional input power. This so-called burning plasma would have a large density of 3.5 MeV energy alpha particles within the ~20 keV plasma. Those alpha particles need to be confined long enough that they deposit most of their energy into the background plasma to keep it at fusion temperature. Without producing large quantities of fusion-alphas, the DIII-D tokamak enables studies of how energetic particles interact with the base plasma using neutral beams to inject 80 keV ions into plasmas with temperatures below 20 keV. Beam ions often excite electromagnetic waves at ion cyclotron frequencies and higher harmonics (5 - 100 MHz) in the 2 Tesla confining magnetic field, and there is renewed interest in turning this emission into a quantitative diagnostic measuring the velocity space distribution of the energetic particles.
Kathreen Thome is advancing the physics that will make such a diagnostic possible. After completing her Nuclear Engineering and Engineering Physics Ph.D. in the Pegasus laboratory at the University of Wisconsin-Madison in 2016, Thome accepted a postdoctoral appointment through Oak Ridge Associated Universities (ORAU) and stationed at DIII-D. While focused on developing steady state plasma scenarios suitable for fusion reactors, she wanted to remain productive in a hands-on way as she was used to from her graduate studies. This work led Thome first to the diagnostics lab, and then to the top of DIII-D where she would repair a set of high frequency magnetic probes to measure these MHz waves.
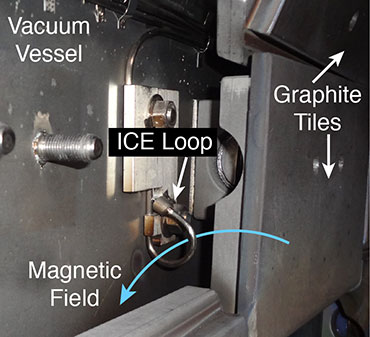
The renewed interest in this physics is due to the fact that the ITER tokamak will produce a large fusion-alpha population that needs to be tracked. While ion cyclotron emission (ICE) had been observed early in the history of tokamak research, complex relationships between ICE and the velocity space distribution of the energetic ions that produce it have largely prevented the ability to convert an ICE observation into a statement about the properties of the ions that produce it. Advances in data acquisition hardware allow for the collecting of magnetic fluctuation data across the 5 - 100 MHz range on a regular basis, regardless of the particular setup of any single DIII-D experiment. Amassing a large database of ICE across different plasma characteristics was exciting enough to convince Raffi Nazikian, Ph.D., a research scientist from the Princeton Plasma Physics Laboratory, to purchase a multi-channel 200 MHz digitizer.
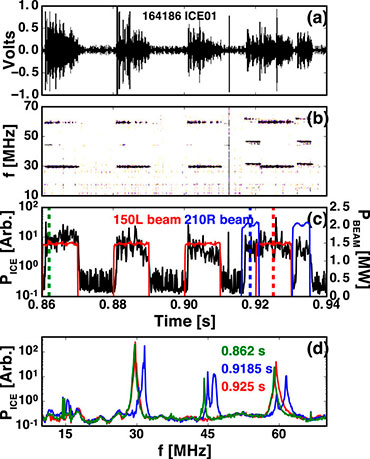
ICE results from K.E. Thome, et al., Review of Scientific Instruments 89, 10I102 (2018)
“We knew that energetic ions must interact with these very high frequency instabilities in DIII-D and we wanted to directly measure these waves for comparison with theory,” said Nazikian, “It is satisfying to see the large volume high quality data generated using the digitizer."
Thome’s task was to resurrect magnetic probes, called ICE Loops, that had not operated for nearly a decade. She brought the system back online with both dedicated magnetic probes and the ability to connect antennas from the Fast Wave Heating system as additional probes. As the photo right shows, some of the ICE Loops are located along the walls of the vacuum vessel behind graphite protection tiles. Another type of ICE Loop is integrated into the tile itself, using the plasma-facing tile surface as part of the measurement loop.
The return of ICE measurements was immediately impactful to the DIII-D team; the system produces 8 - 16 GB of data for each 8 second DIII-D shot, accounting for nearly half of all the data collected on any given shot. The impressiveness of this data collection is not lost on Robert Pinsker, Ph.D., an expert in heating plasmas through electromagnetic wave injection, who also conducts research to determine the meaning of ICE observations.
"The evolution of data acquisition and computer memory equipment over the past few decades has made possible a huge advance in the quantity and quality of ICE data we have been able to collect,” notes Pinsker, “When we started studying this in the late 1980s, we acquired one or a few spectra per shot using an analog spectrum analyzer, with virtually no time resolution and a few kilobytes of data per shot. In 2009 we were able to collect very high time-resolution data, but had enough memory to save only 0.004 seconds of data per shot on two channels. Now we can get microsecond time resolution and save up to 8 channels of data for the whole 8 seconds of the shot! The time has arrived for us to really learn exactly what is going on with these radiofrequency emissions from fusion plasmas."
The physics content is also sizeable, as Thome has identified ICE at frequencies consistent with specific locations of the plasma. For example, high-confinement plasmas (H-modes) typically produce ICE in the far radial edge, while lower confinement plasmas (L-modes) typically produce ICE at frequencies consistent with a core origin. That spatial dependency may indicate the presence of stronger gradients in the energetic ion pressure due to fundamental differences in the plasma density profile. The figure above at right shows that the ICE measurements can also indicate the presence of energetic ion sources by specific particle beams at DIII-D. The raw ICE signals in the top pane show that the amplitude of the magnetic fluctuations get very large corresponding to injections of particle beams (as seen on the bottom panel). Since the beams have different injection geometries, this suggests that the ICE diagnostic system might be able to resolve details of the energetic ion velocity space distribution. As noted by Nazikian, this new data is valuable to theorists who wish to model the expected behavior.
Observations of ICE at frequencies corresponding to the core of the tokamak are particularly interesting for applications to ITER. There, the fusion alpha population will be core-localized. Ongoing work will provide some of this new data to theorists who can improve ICE theory such that an ICE diagnostic can be developed for ITER. A joint experiment of the International Tokamak Physics Activity (ITPA) Energetic Particle Physics Topical Group is pooling together experimental and theoretical results from fusion facilities around the world to further advance this topic. Thome is excited to contribute her efforts to this cause, as she notes, “Impending ITER plasmas and the recent capability of fast digitization is creating a renaissance of interest in ICE data that makes it a fulfilling topic to research.”
This work is supported in part by the U.S. Department of Energy, Office of Science, Office of Fusion Energy Sciences, using the DIII-D National Fusion Facility, a DOE Office of Science user facility, under Awards DE-FC02-04ER54698 and DE-AC02-09CH11466.
Disclaimer: This report was prepared as an account of work sponsored by an agency of the United States Government. Neither the United States Government nor any agency thereof, nor any of their employees, makes any warranty, express or implied, or assumes any legal liability or responsibility for the accuracy, completeness, or usefulness of any information, apparatus, product, or process disclosed, or represents that its use would not infringe privately owned rights. Reference herein to any specific commercial product, process, or service by trade name, trademark, manufacturer, or otherwise does not necessarily constitute or imply its endorsement, recommendation, or favoring by the United States Government or any agency thereof. The views and opinions of authors expressed herein do not necessarily state or reflect those of the United States Government or any agency thereof.
Additional Information
DIII-D National Fusion Facility - https://fusion.gat.com/global/diii-d/home
ITER - https://www.iter.org/
Princeton Plasma Physics Laboratory - http://www.pppl.gov/
U.S. Department of Energy, Office of Science, Office of Fusion Energy Sciences - https://science.energy.gov/fes/
International Tokamak Physics Activity (ITPA) Energetic Particle Physics Topical Group - https://www.iter.org/org/team/fst/itpa/ep
K.E. Thome, et al., “Radio Frequency Measurements of Energetic-Particle-Driven Emission using the Ion Cyclotron Emission Diagnostic on the DIII-D tokamak,” Review of Scientific Instruments 89, 10I102 (2018) https://aip.scitation.org/doi/10.1063/1.5035561
K.G. McClements, et al., “Fast particle-driven ion cyclotron emission (ICE) in tokamak plasmas and the case for an ICE diagnostic in ITER,” Nuclear Fusion 55, 043013 (2015) https://doi.org/10.1088/0029-5515/55/4/043013